Quantum entanglement is one of those mind-blowing concepts in science that shows just how deeply connected everything can be. Imagine two particles so closely linked that when something happens to one, the other reacts instantly — no matter how far apart they are. Even though this sounds like science fiction, countless experiments prove it’s real. Einstein himself found it so odd that he called it “spooky action at a distance” because it seemed to defy the laws of physics as we know them.
Today, scientists are exploring all kinds of uses for quantum entanglement, from super-fast computers to secure communication systems that can’t be hacked. It’s even opening up exciting possibilities for health and wellness. Quantum-based services, like Quantum Upgrade, aim to help people connect to quantum energy fields to boost energy, improve well-being, and even support cellular health.
So while we’re still uncovering the mysteries of entanglement, it’s clear that this strange, fascinating connection between particles might just be the key to transforming technology, health, and our understanding of the universe itself.
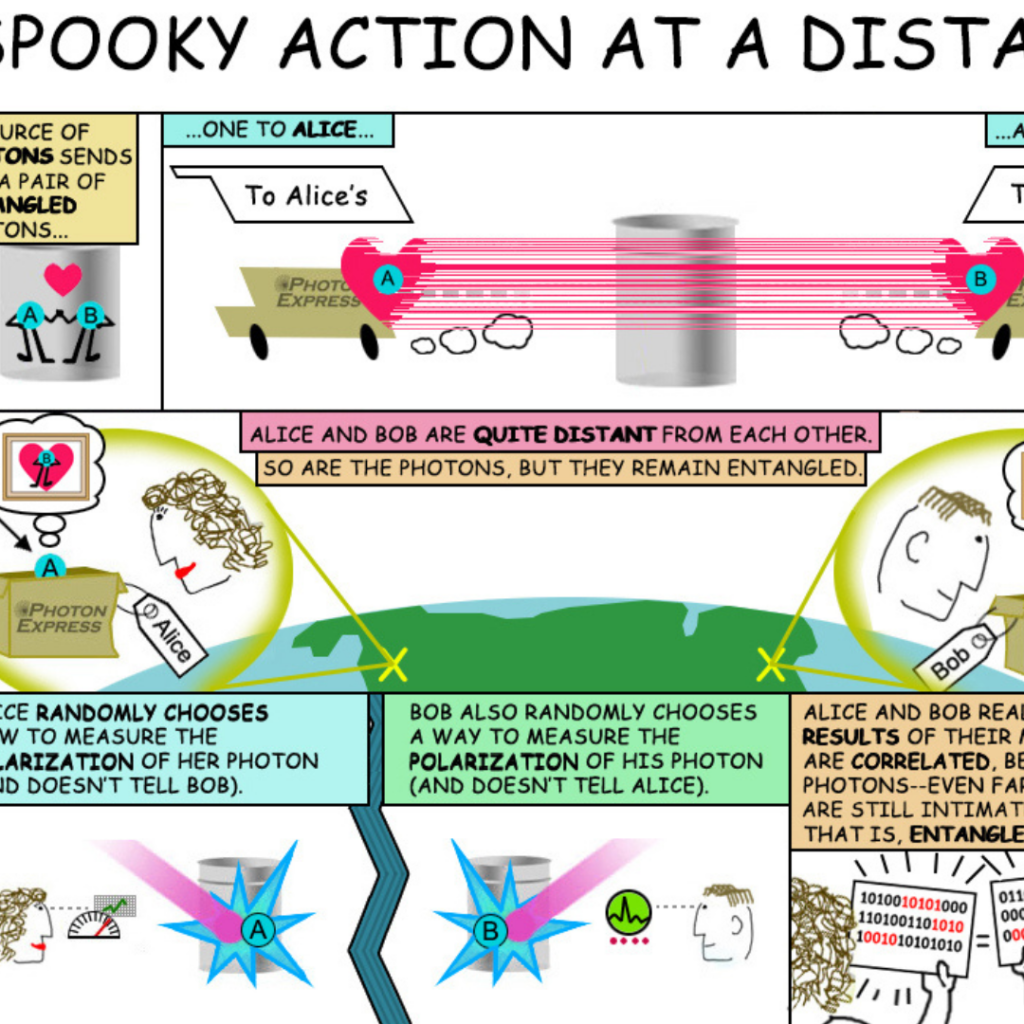
What Is Quantum Entanglement?
Quantum entanglement is a phenomenon in quantum physics where two or more particles become interconnected. The state of one particle is directly related to the state of the other, no matter how far apart they are.
If you measure one particle’s properties (such as spin, position, or momentum), the properties of the entangled partner(s) will be instantly known or influenced, even if they are separated by vast distances.
In a video produced for the Caltech YouTube channel, professor of physics and Rosenberg Scholar Manuel A. Endres explains that entangled particles are always correlated. He says that “entanglement is just correlation on a quantum level.” When Albert Einstein discussed quantum entanglement as part of the EPR Paradox experiment, he was skeptical and dismissed it as “spooky action at a distance.”
Here’s a breakdown of key aspects of quantum entanglement:
- Interconnectedness across distance: When particles are entangled, changes made to one particle affect the other instantaneously, regardless of the distance between them. This instantaneous connection appears to violate classical notions of time and space, where such communication would be limited by the speed of light — however, quantum entanglement isn’t faster than the speed of light, which we’ll explain in more detail below.
- Non-Locality: Quantum entanglement suggests a form of “non-local” interaction, meaning that the particles are not bound by the usual constraints of physical proximity. This non-locality puzzles scientists because it seems to defy the rules of classical physics.
- Measurement correlation: Once the state of one particle in an entangled pair is measured, the state of the other particle is immediately determined. For instance, if two particles are entangled in a way that their spins are opposites, measuring the spin of one particle will instantly reveal the spin of the other, even if they are light-years apart.
One odd thing to note about measurement of quantum entangled particles, is that the act of measuring itself affects the results. So if you measure one particle and find its spin is up, its partner’s spin will be down. But if you go back and measure the first particle again, there’s only a 50% chance that its spin will be up…even though that was the result the first time you measured it.
The EPR Paradox
Quantum entanglement was famously discussed by Albert Einstein, Boris Podolsky, and Nathan Rosen in a thought experiment known as the EPR paradox. They used this paradox to argue that quantum mechanics must be incomplete because it allowed for spooky action at a distance, the phrase Einstein coined to express his discomfort with entanglement. Later, experiments confirmed that quantum entanglement does occur, solidifying it as a key feature of quantum theory. We’ll discuss some of these experiments later.
Did Einstein Believe in Quantum Entanglement?
Einstein was uncomfortable with the idea of quantum entanglement, specifically the non-locality part. Measuring a particle and instantly knowing its entangled partner’s state was troubling for Einstein, and why he called it “spooky.” He thought there must be some “hidden variables” to explain what was happening.
Einstein was one of the first scientists who tried to understand quantum entanglement, according to Raymond Laflamme, OC, FRSC, associate faculty member at the Perimeter Institute for Theoretical Physics. “He wanted to show that it [quantum entanglement] was not part of our world,” says Laflamme in a video on the Perimeter Institute’s YouTube channel. “It took another 29 years before an Irish physicist, John Bell, kind of turned his [Einstein’s] ideas into an equation.”
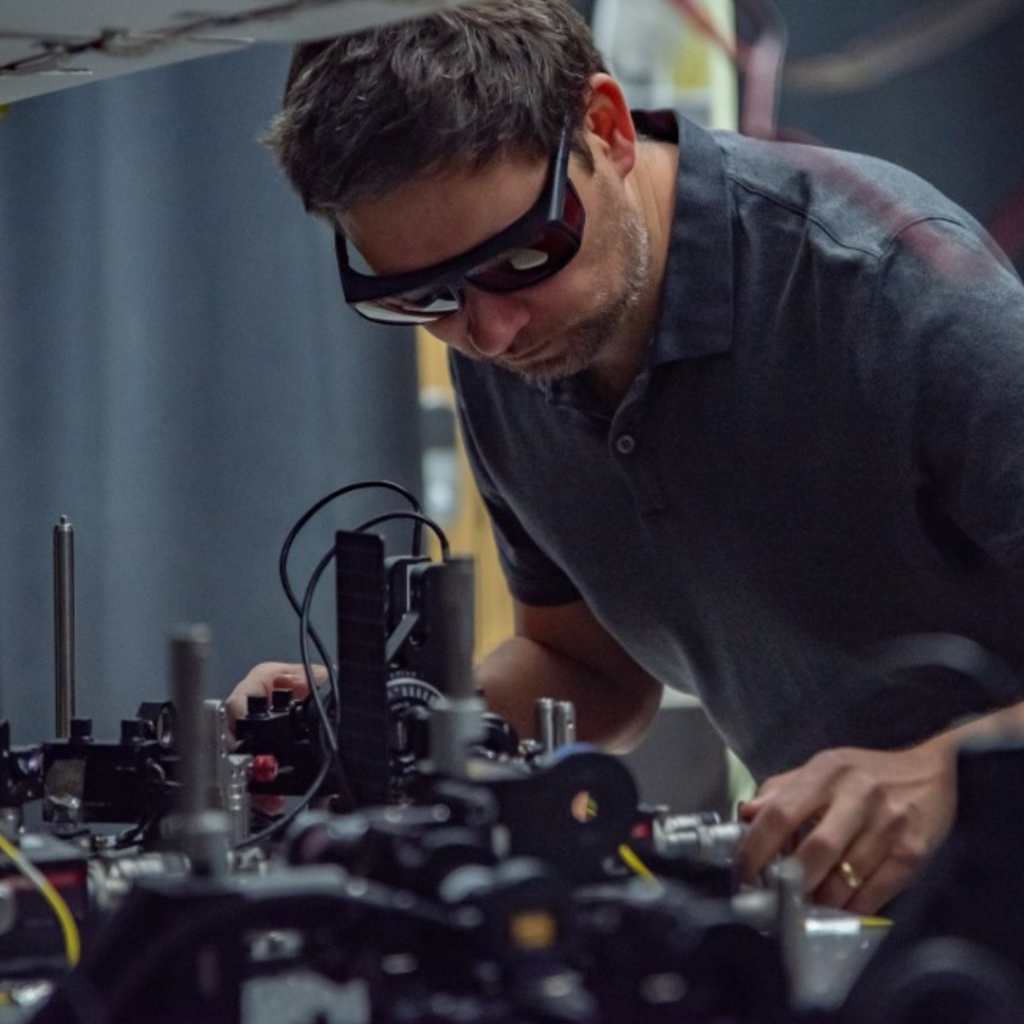
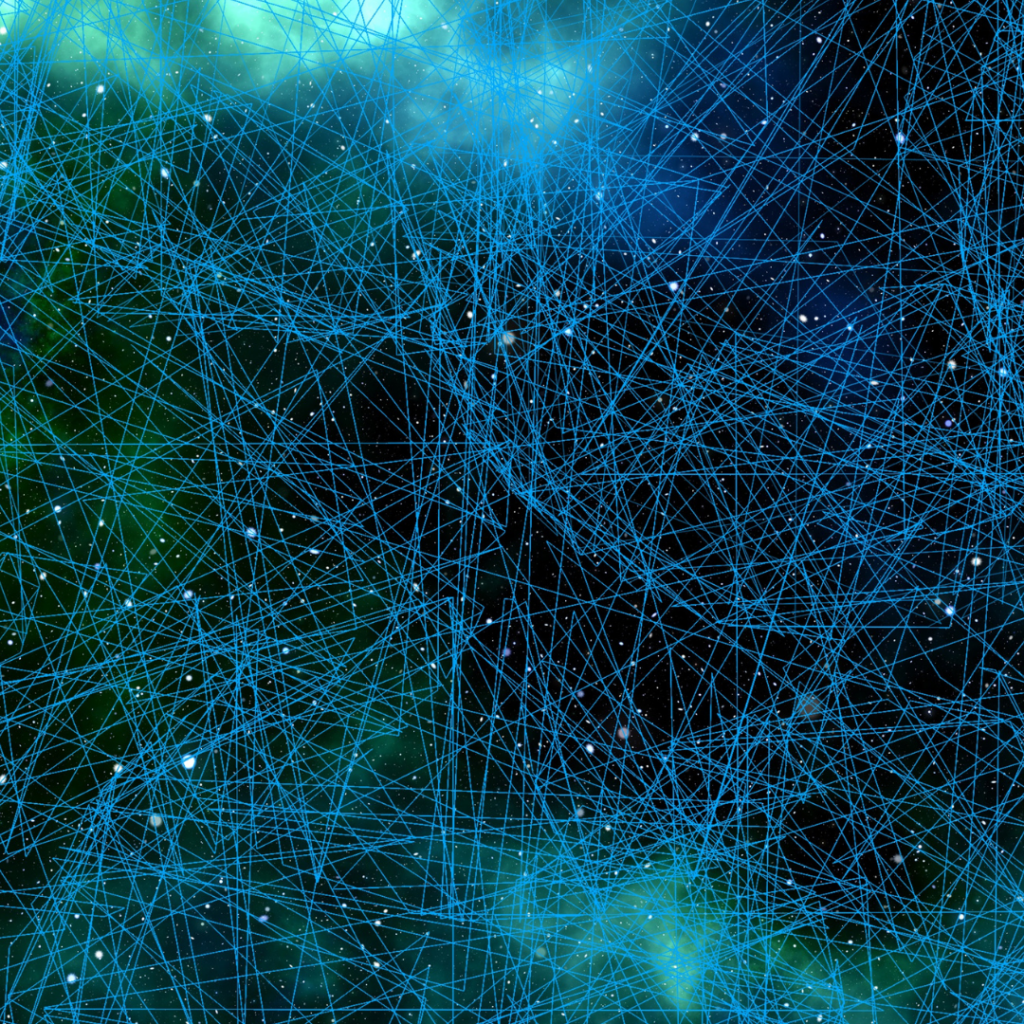
Then, in 2022, Alain Aspect, John F. Clauser, and Anton Zellinger won the Nobel Prize for Physics for proving Bell’s theory and demonstrating that unseen particles can be entangled with each other even when separated by large distances. Their work proved Einstein wrong.
Other Tests Proving Quantum Entanglement
In addition to the 2022 experiments that proved John Bell’s theory, there were also three papers published in 2015 that supported Bell’s hypothesis.
One of these papers was published by physicist Krister Shalm and his team at the National Institute of Standards and Technology (NIST). In their experiment, they were able to see how the measurements of one photon affected the other photon in an entangled pair.
In a statement on NASA’s blog, the paper’s co-author Francesco Marili (of NASA’s Jet Propulsion Laboratory) said, “Our paper and the other two published last year [2015] show that Bell was right: any model of the world that contains hidden variables must also allow for entangled particles to influence one another at a distance.”
Technically, Quantum Entanglement Isn’t Faster than Light
Because of the nature of quantum entanglement, some think that it represents information traveling faster than the speed of light. However, entangled particles cannot do faster-than-light communication. There are a few key reasons why.
Instantaneous Correlation vs. Information Transfer
Entangled particles have instantaneous correlation — but that doesn’t mean they can communicate faster than light. When you measure the state of an entangled particle, the outcome is random and cannot be controlled. For example, if two particles are entangled and particle A is measured as spinning up, particle B will be spinning down. They’re always correlated. But knowing that particle A’s spin will always be opposite to particle B’s spin doesn’t let you know what particle A’s spin is. Particle A’s spin is random and unpredictable.
While you might know what particle B’s spin is as soon as you measure particle A, you can’t tell your colleague at the particle B measuring station what your measurement results were any faster than the speed of light can travel. So although it seems that information about one particle’s state is instantly available upon measuring the other, no actual information transfer occurs.
Thomas Vidick, a professor of computing and mathematical sciences at Caltech, explains in an article on the Caltech blog Science Exchange, “There can be correlation without communication,” and the particles “can be thought of as one object.”
Trying to use entangled particles to send information faster than light essentially forces one of the particles out of its quantum state and makes the two no longer entangled, according to physicist and science writer Ethan Siegel. In an article for Big Think, he explains, “Quantum entanglement can only be used to gain information about one component of a quantum system by measuring the other component so long as the entanglement remains intact. What you cannot do is create information at one end of an entangled system and somehow send it over to the other end.”
Therefore, the two particles being correlated doesn’t equate to them communicating with each other. Which means that quantum entanglement technically doesn’t violate Einstein’s Theory of Relativity.
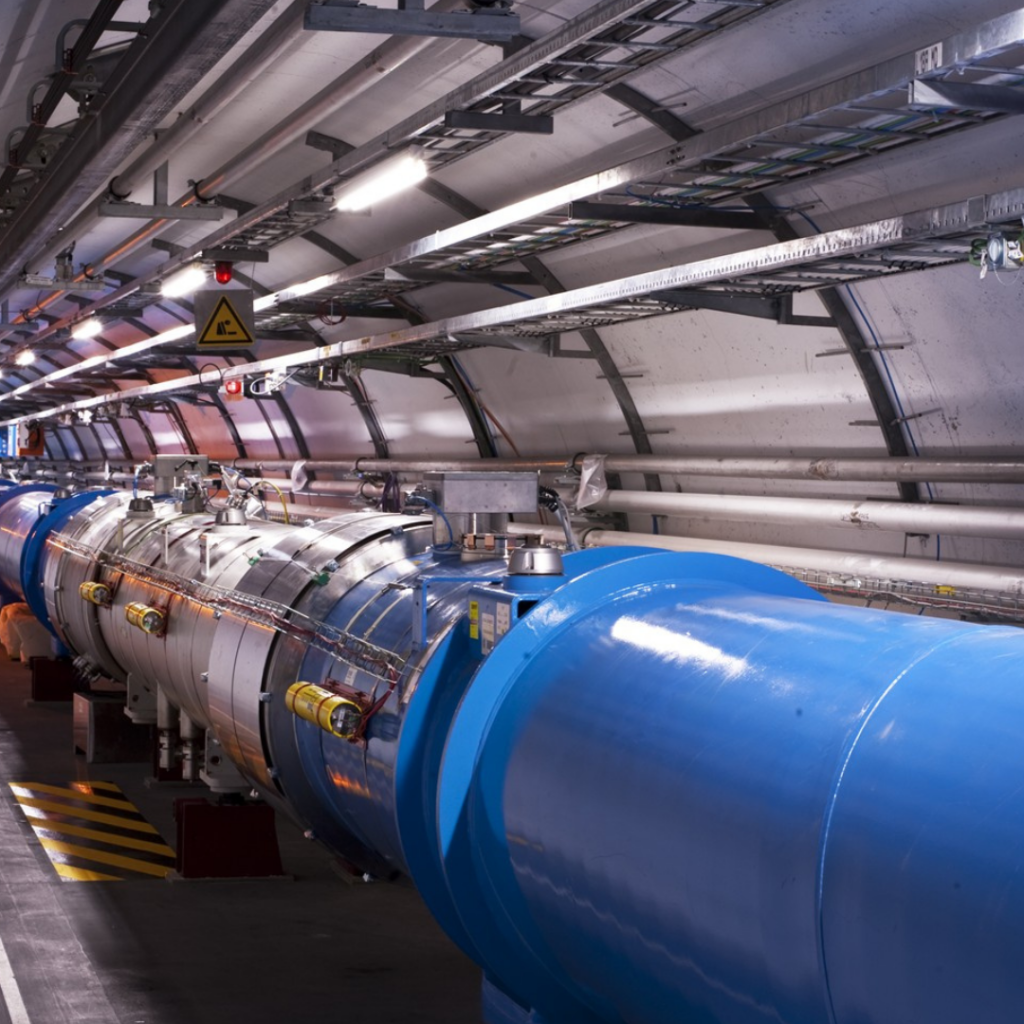
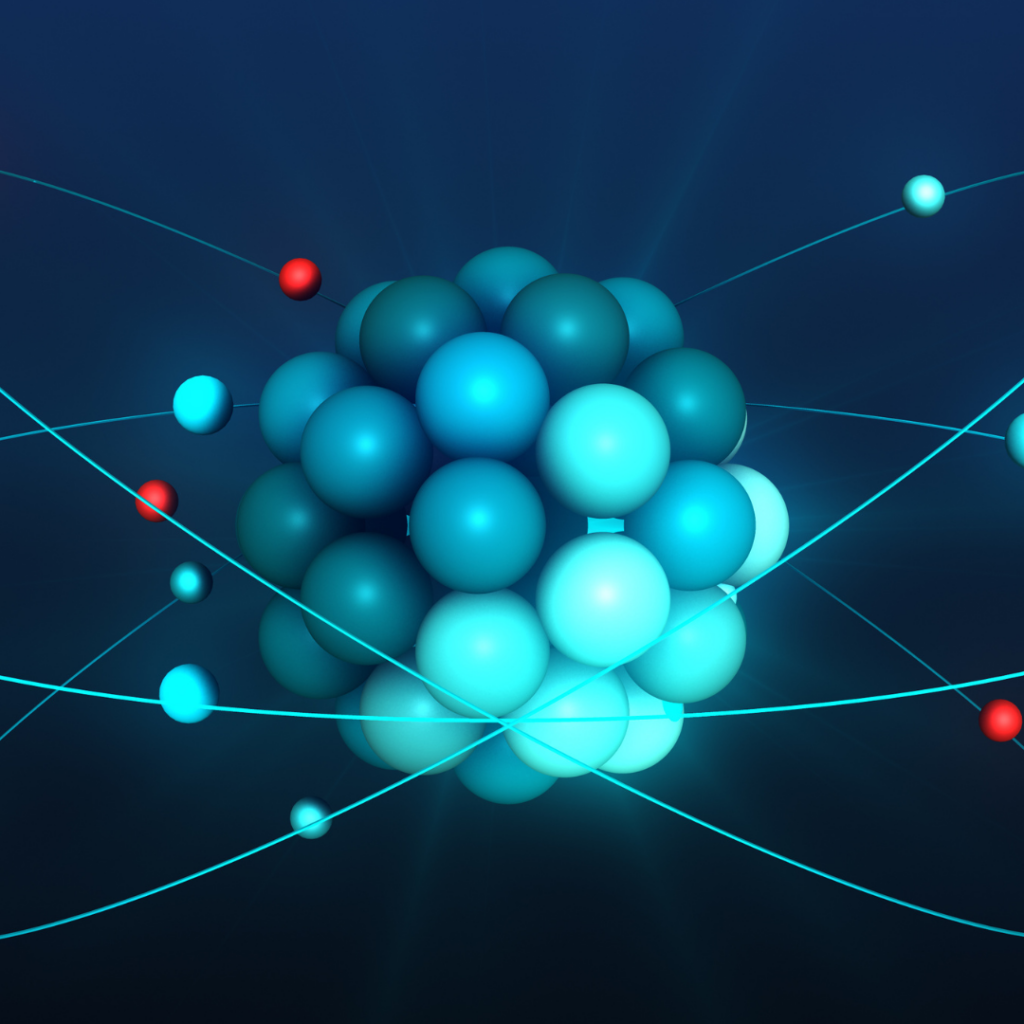
Causality and Relativity
The principles of relativity dictate that no information can travel faster than light without violating causality—the cause-and-effect relationship fundamental to physics. Allowing faster-than-light communication would lead to paradoxes where an effect could precede its cause.
This principle has been upheld in numerous theoretical explorations and experiments since Einstein’s formulation of relativity, such as Aspect’s experiment in 1982 and the relativistic particle collisions with CERN’s Large Hadron Collider starting in 2008.
How Two Things Become Quantum Entangled
Most experiments with quantum entanglement use photons, since photons are easy to entangle. According to Chad Orzel, Associate Professor in the Department of Physics and Astronomy at Union College, there are generally five ways you can create quantum entanglement:
1. Collision Cascade
A collision cascade in quantum physics is a series of energetic collisions between atoms in a solid or liquid material, initiated by a high-energy particle. It’s triggered by an energetic particle, such as an ion from a particle accelerator, a recoil atom from a high-energy neutron, electron, or photon, or an atom recoiling from radioactive decay. Photons emitted through a collision cascade must have polarizations correlated to each other — in other words, they must be entangled.
2. Spontaneous Parametric Down-Conversion
The spontaneous parametric down-conversion (SPDC) process involves a nonlinear crystal where a single photon is split into two lower-energy entangled photons. When a high-energy photon enters the crystal, it can be converted into two photons that are entangled in terms of their polarization states.
3. Second-Generation Entanglement
This method involves getting a pair of entangled photons to absorb into a pair of atoms, so that the atoms become entangled. Why bother with this process? Although photons are easy to entangle, they are always constantly moving at the speed of light. Entangling and measuring material particles is useful because you can hold on to them for longer periods of time. Orzel admits that this process is tricky, however.
4. Entanglement by Accident
This method involves getting two atoms that emit photons, collecting the photons, and then bringing them together on a beamsplitter with two photodetectors placed at the two outputs of the beamsplitter. Photons will reach the beamsplitter about 25% of the time, and when one photon is detected at each output, they have opposite polarizations, i.e. they’re entangled.
5. Entanglement by Interaction
Particles can become entangled by letting them interact directly with each other. A good example of this is the “Rydberg blockade,” which involves two atoms that don’t normally affect each other when they’re in a low-energy state. But when you give one of the atoms extra energy (putting it in what’s called a “Rydberg state”), they start to interact. If you use a laser to put the first atom in a mix of both low-energy and high-energy states (called “superposition”), and then try to excite the second atom, the two atoms end up in opposite states, or entangled.
Orzel notes that all these methods of entangling particles are local, meaning the two particles are either in the same place at some point or they interact when something passes between them. He says that this aspect “is a critical feature for keeping the weirdness of entanglement contained — you can’t just arbitrarily entangle two particles that have no common history.”
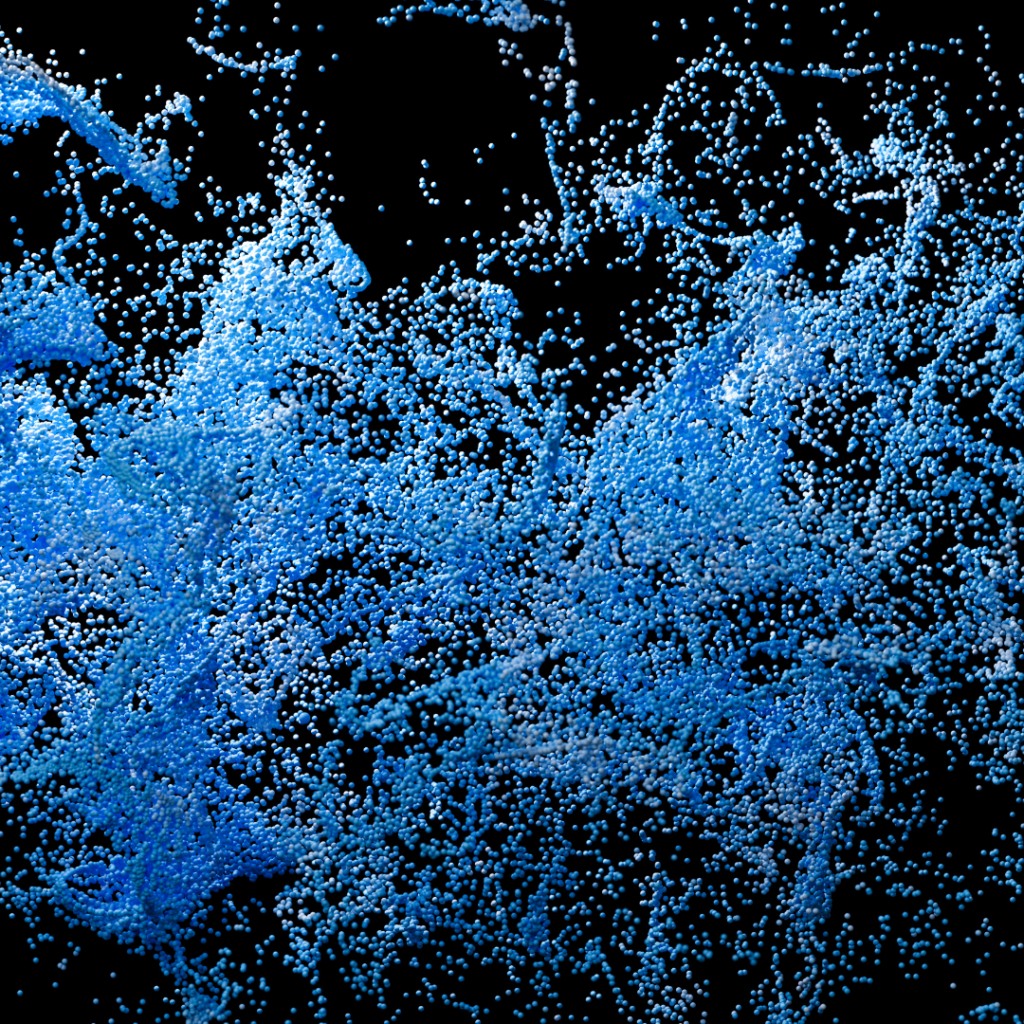
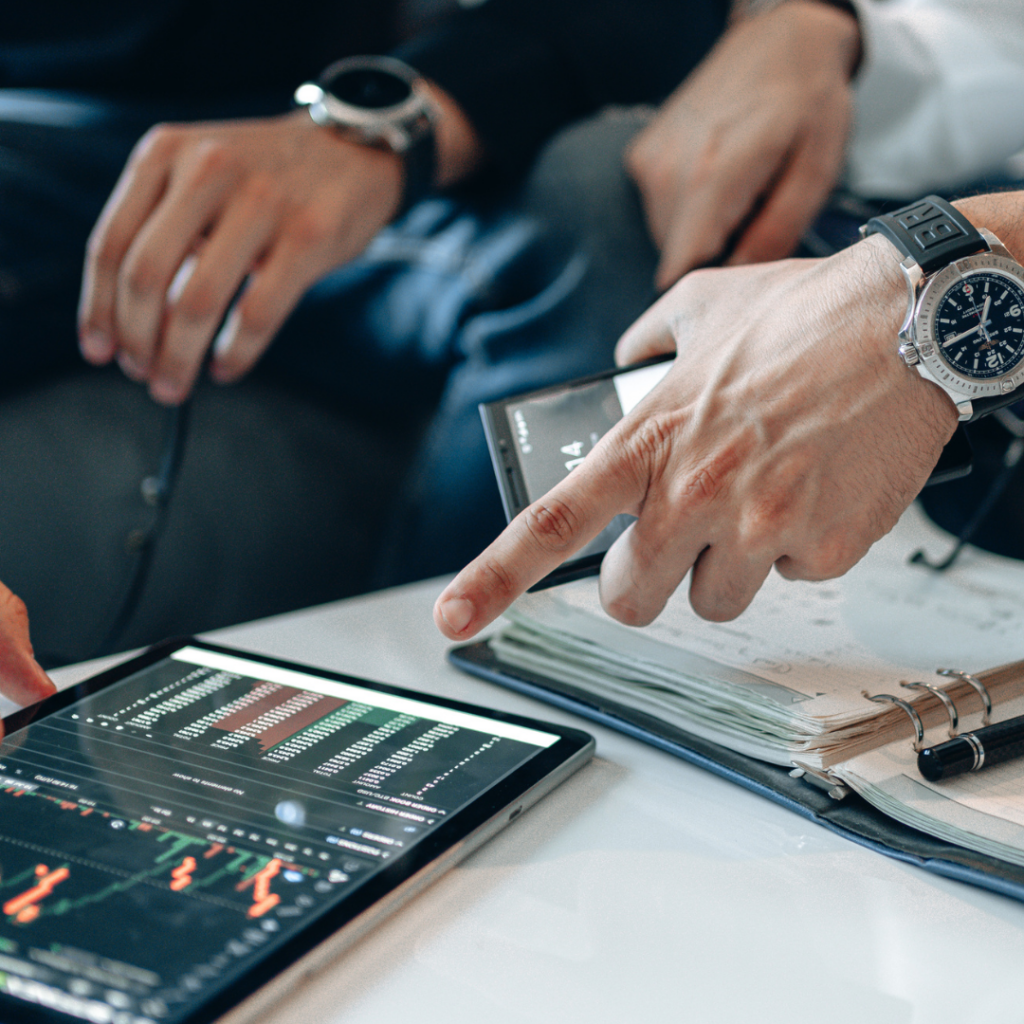
Quantum Entanglement Happens Naturally
All the methods for creating quantum entanglement listed above involve laboratories, special equipment, and human intervention. So, can quantum entanglement happen naturally? Of course! When two things interact, they entangle. Particles are getting entangled or being created as entangled in nature all the time.
However, many people wonder if quantum entanglement as it exists in nature can be studied, since quantum systems are so small and require specialized equipment to detect. It’s completely natural, and we may even be able to see it with the naked eye. A study in 2011 showed that entangled diamonds vibrated together.
Here are some other examples of quantum entanglement in nature:
- Subatomic particles: When a particle decays into multiple particles, those multiple particles are entangled.
- Photon emission: When an electron or atom emits two photons, those photons are typically entangled.
- Biological systems: Entanglement might be present in certain biological processes, such as certain cells showing effects of radiation even when they haven’t been directly targeted by it, suggesting signaling or entanglement between cells.
- Large-scale systems: Entanglement can involve millions of particles, such as with “strange metals” (metals whose electrons can become quantum entangled with one another) and novel superconductors.
Quantum entanglement occurs so often that you could say it’s one of the most natural things in the universe. When Einstein and his colleagues first studied quantum entanglement in the 1930s, he found it “spooky” and considered it unnatural. Since then, we’ve come to realize this is not the case at all.
“Einstein wanted all of nature identified with a simple and compact classical description,” said Chris Ferrie, author and faculty member of the Center for Quantum Software and Information at the University of Technology Sydney in his article for Scientific American. He went on, “But we now know that quantum information provides the most accurate description of nature, which is written in a language we do not speak.”
Practical Uses for Quantum Entanglement
Quantum entanglement is more than just a theoretical curiosity. It plays a key role in the development of quantum computing, quantum cryptography, and quantum teleportation. In quantum computers, for instance, entanglement allows for complex calculations to be performed simultaneously, vastly increasing processing power.
In quantum cryptography, entanglement ensures that data can be transmitted securely, as any attempt to intercept the information would alter the quantum states and be detectable.
Quantum entanglement can also help facilitate deep-space communication. It could allow for higher bandwidth communication over vast distances where signal strength is limited. There’s even potential for a future “quantum internet” that links Earth-based and space-based quantum devices.
We could also take more high-precision measurements with entangled particles by creating quantum sensors with unprecedented accuracy for measuring physical quantities like time, gravity, and magnetic fields. In the same vein, entanglement could also make atomic clocks (which power GPS and other timing-dependent technologies) more precise.
You can also harness the power of quantum entanglement and quantum energy to enhance well-being and increase energy levels. Quantum fields are all around us, bringing matter into form and shaping reality. Your body interacts with these fields, either by receiving or losing energy.
One way to support the body’s connection to quantum fields and maximize the positive energies surrounding you is with our Quantum Upgrade service. It uses quantum entanglement to facilitate instantaneous energy transfer, even across distances, which can uplift and energize any area of your life. It’s a unique way to tap into the non-linear power of quantum energy for lasting, positive transformations.
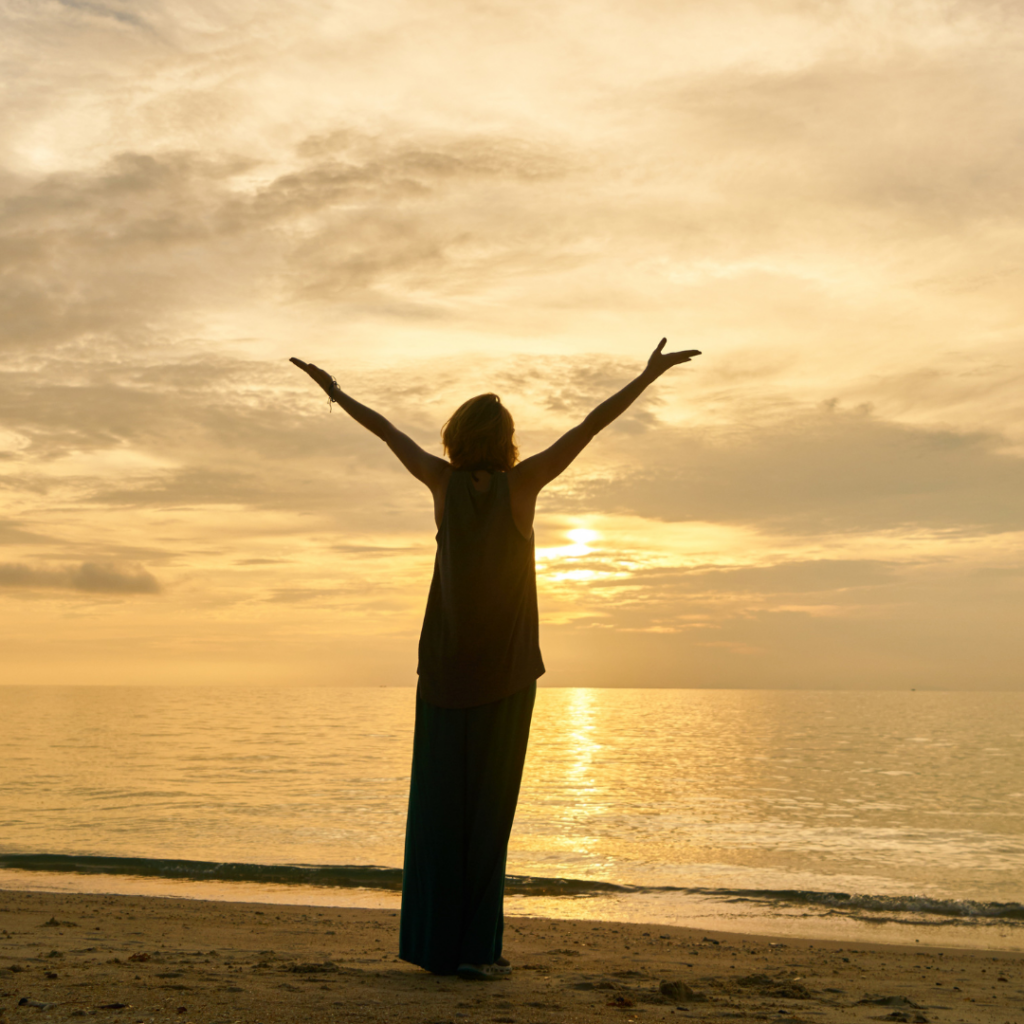
Conclusion: The Exciting Future of Quantum Entanglement
Scientists are the first ones to admit that quantum entanglement doesn’t quite make sense. They know it exists and they’ve proven it with several experiments, but they’re still trying to figure out why it works the way it does. Physicists stop short of calling it magic — they are scientists after all — but they don’t deny that what we know about quantum entanglement today would very possibly have been considered magic 50 years ago.
As scientists continue to unravel the mysteries of quantum entanglement, its potential applications are expanding beyond physics and into fields like health and technology. Some researchers are exploring how quantum energy may impact the human body, potentially enhancing energy flow, supporting cellular health, and improving how the body manages stress. These studies could open new avenues for medical innovation, offering ways to harness this invisible force for well-being and healing.